The Vital Link: How HVDC Is Modernizing the Grid
Credit to Author: Sonal Patel| Date: Wed, 01 Jun 2022 04:16:00 +0000
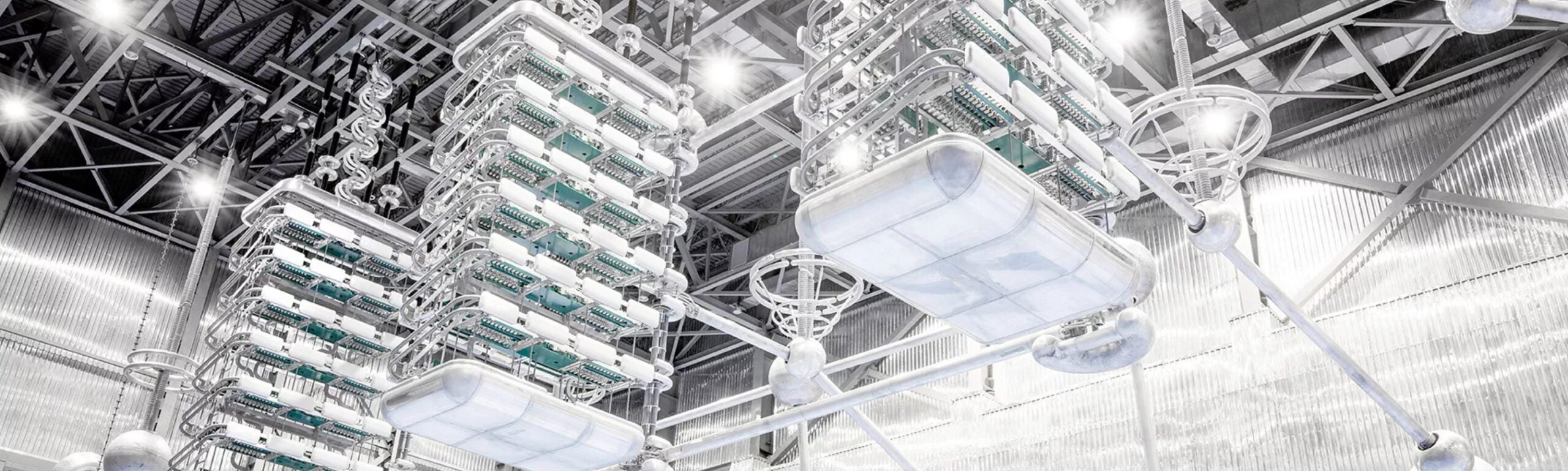
Significant advances in high-voltage direct current (HVDC) transmission are in step with rapid changes to energy systems worldwide.
Shortly after POWER magazine began publication in 1882, the competitive technology battle between Thomas Edison and Nikola Tesla—the legendary “War of the Currents”—began to brew. Edison had developed direct current (DC), which essentially runs continually in a single direction like a battery or a fuel cell, allowing early generating stations to deliver electricity over copper wires. Though revolutionary, the method was so inefficient, power plants remained tethered within a mile of the load they served. And though DC was widely adopted, it was not easily converted to higher or lower voltages.
Tesla posited alternating current (AC) was a better solution. Reversing direction a certain number of times per second (60 in the U.S.), AC could be converted to different voltages relatively easily using a transformer, enabling the transmission of power over long distances using smaller wires at a higher voltage. AC for the most part proved to be the more cost-effective and flexible high-voltage transmission solution, and it was quickly adopted as a hallmark of power networks everywhere.
The War of the Currents was not definitively won, however. Attempts to transform DC voltage to higher or lower levels continued. In 1954, after some marked demonstration triumphs, Swedish engineering firm ASEA opened the world’s first high-voltage direct current (HVDC) link between mainland Sweden and the island of Gotland (Figure 1), based on a 1929-patented low-pressure mercury-arc converter designed by Dr. Uno Lamm (who is largely considered the “Father of HVDC”).
![]() |
1. In 1954, ASEA, the predecessor of ABB, used this classic technology utilizing mercury arc valves to construct the world’s first commercial high-voltage direct current (HVDC) link between Västervik, on the east coast of Sweden, and Ygne, on the island of Gotland in the Baltic Sea. The original Gotland link could transfer 20 MW over a 98-kilometer-long submarine cable with a voltage of 100 kV. Courtesy: Hitachi Energy |
Advancements came fluidly thereafter. In 1957, General Electric introduced silicon-controlled rectifiers (now known as thyristors), a more efficient solid-state semiconductor converter technology. While thyristor valves have ultimately replaced many of the HVDC lines with mercury valves (which are known as line-commutated converters), thyristor valves still require an external AC circuit to turn them off or on.
In the 1990s, ABB, a company that evolved from ASEA, pioneered a voltage source converter (VSC) under its HVDC Light brand, and subsequently installed the world’s first VSC link in Gotland. VSCs, which are more compact than previous converters, ushered in a new HVDC capability (see sidebar) to switch on and off many times to improve harmonic performance, without being dependent on synchronous machines in the AC system for its operation.
Why HVDC?Most high-voltage direct current (HVDC) lines are point-to-point lines that typically carry power, unidirectionally and in a controlled manner, from a large power plant or agglomeration of power plants to a single converter station in a distant alternating current (AC) grid. An HVDC link typically comprises its generation sources (except in intertie applications), AC transmission lines (to provide a dedicated collector system), an AC to DC converter station, HVDC transmission lines, a DC to AC converter station, AC transmission and distribution lines, and, finally, its end-users. “An AC cable and a DC cable have a pretty large electrical capacitance,” or the ratio of the amount of electric charge stored on a conductor to a difference in electric potential, Roger Rosenqvist, vice president of business development for Hitachi Energy’s HVDC division in North America, explained to POWER in May. “And so, if you connect an AC cable to the grid, before you even start to push any power through that cable, you will see a pretty high current in the cable because it’s constantly charged and recharged with the power frequency. At a certain critical length, you have essentially used up the whole capacity rating of the cable just for the charging current, [leaving] no room to transmit real power in the system,” he said. “When you want to increase the capacity rating on an electrical circuit, you typically do that by going to higher voltage, but when you go to a higher voltage on an AC cable, you increase the charging current. So, a high-capacity AC circuit needs to go to a high voltage like 345 or 500 kV, but a 500-kV cable has a shorter practical distance than a 345-kV cable or a 230-kV cable.” And while AC circuits can transmit power at higher distances at lower voltages, that is compounded with the associated reduced capacity in the cable, which often requires multiple cables. “So, there is a maximum length at which you can transmit electric power in the submarine or underground cable using AC technology,” he said. For DC, “you charge the electrical capacitance of the cable when you energize it, but then the voltage stays constant, so there is no routine charging and recharging of the cables,” Rosenqvist said. “Once a DC cable has been energized, you have the capacity of the electrical conductor in the cable available for transmitting real power, and there is really no practical length limitation on how far you can go with a DC cable.” DC cables can enable transmissions of up to 2,000 MW at very high voltages in a single circuit, “which is not possible using AC technology,” Rosenqvist said. |
The Evolving Technology Landscape
Currently, two main HVDC technologies dominate the landscape: line-commutated converters (LCC), also known as current-source converters (CSC) using thyristors, and VSC, using insulated-gate bipolar transistors (IGBT). Experts suggest that for long-distance and high-capacity transmission links, CSC-HVDC technology has both higher efficiency and a higher power transfer capacity than classical AC solutions. But for power transmission from offshore power plants or from other remote areas with limited space, VSC-HVDC is the preferred technology (Figure 2), owing to its smaller footprint, and active and reactive power control capabilities.
![]() |
2. Hitachi Energy, a joint venture firm officially launched in 2020 when Japanese technology conglomerate Hitachi acquired a majority share in Swiss conglomerate ABB’s Power Grids business, says 70% of the world’s 200-GW installed HVDC base is installed with its technology. This includes half of all voltage-source converters (VSC) in the world. Courtesy: Hitachi Energy |
VSC’s success over the past 10 years may point to a larger role in the ever-more complex power systems landscape, said Roger Rosenqvist, vice president of business development for Hitachi Energy’s HVDC division in North America. Combined with underground transmission using polymer insulated cables “there has been a scaling up of the capacity of VSC, and today we can build 3,000-MW systems,” which has opened up new opportunities, he said.
However, several other technologies are also emerging to provide innovative solutions to long-standing capability gaps, such as modular multilevel converters (MMC), cascaded two-level converters (CTL), and hybrid VSC-CSC links. MMC, a technology first proposed in 2003, is a VSC converter that has two forms: “half-bridge” and “full-bridge.” MMC technology was commercially deployed for the first time worldwide in 2018 as an upgraded control system in an HVDC system in the Trans Bay Cable (TBC) project (Figure 3), which includes a 53-mile HVDC submarine cable that runs under San Francisco Bay.
![]() |
3. Siemens Energy in 2018 unveiled an upgraded control system for the San Francisco Trans Bay Cable project, an HVDC transmission system with VSC technology realized in a modular multi-level converter (MMC) topology. Courtesy: Siemens Energy |
The project was notably Siemens Energy’s first successful MMC converter test for black-start capability using load banks. “Practically speaking, each module within an MMC is a discrete voltage source with a local capacitor to define its voltage step without creating ripple voltage distortion across the converter’s other phases,” says Siemens Energy. “This way it is possible to achieve the required sinusoidal AC and smooth DC side output voltage waveforms without excessive harmonic distortion and [high-frequency] noise.”
According to the European Network of Transmission System Operators for Electricity (ENTSO-E), MMC “is becoming the preferred power electronic converter for converting between AC and DC as it presents several benefits.” Along with the ability to reverse the power flow without reversing the polarity of the DC voltages by DC current reversal, MMC is modular and scalable, “making it advantageous compared to other VSC topologies,” ENTSO-E said. MMC also has an inherent capability of storing energy internally in the converter. “This can benefit the system in which it is connected and enables the drastic reduction of operating losses of the converter stations by avoiding the need for high-frequency switching of the semi-conductor devices,” the grid organization noted.
Significant Applications for a Rapidly Evolving Grid
HVDC’s suitability to the rapidly changing grid, meanwhile, is pegged to its many advantages over classical AC solutions, such as active power control, higher transfer capacities, no reactive power, longer possible transmission lengths, and lower active power losses. According to some experts, in some cases, it’s the only feasible technology.
Long-distance bulk power transmission, with the added benefit of cost-effective and efficient transmission, continues to be HVDC’s most shiny attribute. Several lengthy lines have been built in recent years. A much-cited example is the 2018-completed Changji-Guquan Link, a CSC–HVDC project that can transfer 12 GW at 1,100 kV over 3,324 kilometers (km)—stretching from China’s far northwest to the heavily populated east. According to Hitachi Energy, the link offers a 50% increase in transmission capacity compared to the 800-kV ultra-high direct current (UHDC) links in operation.
State Grid of China, the line’s operator, reported the project has strengthened the outbound delivery capacity of the Xinjiang Power Grid, as well as promoted development of the Xinjiang Uygur autonomous region, which is rich in coal, wind power, and solar power. The project remains the world’s record holder in terms of voltage level, transmission capacity, and distance. A similar project in Germany, SuedOstLink, is poised to transport wind power from the north to consumers in the southern regions (Figure 4).
![]() |
4. Cable systems manufacturer Prysmian Group in December said it produced the first 20 kilometers (km) of underground cable for the SuedOstLink. This image shows the first lots of cables—a total of 9 drums, each with 1,750 meters of underground cable. Prysmian will produce a total of about 550 km of underground cable for grid operator TenneT for an approximately 270 km long section of the SuedOstLink (starting at the Southern Germany connection point at Isar, close to Landshut in Bavaria). For this project two HVDC underground cables will be laid in one trench. Courtesy: Prysmian |
But HVDC is also well-suited to submarine cable transmission at distances beyond 50 km, because HVDC submarine cables do not have the physical restrictions AC cables have, owing to the reactive power component. This allows underwater lines to link to asynchronous networks, connect offshore wind power, or supply remote islands. The October 2021–commissioned 720-km North Sea Link, for example, harnesses Norway’s hydropower and the UK’s wind energy resources, providing a new measure of flexibility for the two countries’ power grids. The 1,400-MW VSC (HVDC Light) link is today the longest subsea connector in the world.
Another application that is quickly gaining traction is HVDC’s ability to interconnect networks that operate asynchronously. According to Rosenqvist, that feature might be especially beneficial in North America, where many asynchronous interconnections exist, such as between the Eastern Interconnection and Western Interconnection systems, and between the Electric Reliability Council of Texas (ERCOT) and neighboring grids. One project that could highlight this HVDC benefit is New York’s newly approved $4.5 billion Champlain Hudson Power Express, an underwater and underground power cable project linking Quebec to New York City. That 546-km line is expected to be completed in 2025.
Finally, the burgeoning landscape of offshore wind power has begun an effort to harness VSC’s independent control capabilities of both active and reactive power flow, the ability of “black-start,” as well as its low-ground footprint. Several projects in the North Sea have chosen HVDC connections. That trend will carry over to the U.S., owing mainly to its deeper water installations, said Rosenqvist.
![]() |
5. A consortium of Siemens Energy and Aker Solutions in October 2021 won a contract to supply the HVDC system that will bring offshore wind power from Ørsted and Eversource’s 924-MW Sunrise Wind farm, one of New York’s first utility-scale offshore wind projects. Courtesy: Siemens Energy |
“There’s interest in HVDC for offshore wind integration in the Northeast, whereas, the very first wave was typically with AC connection because the wind resource lease areas were a little closer to the coast,” he said. America’s first offshore HVDC project, a 320-kV, 161-km line, could be deployed by Siemens Energy and Aker Solutions for Ørsted and Eversource’s 924-MW Sunrise Wind project in New York by 2025 (Figure 5).
According to Rosenqvist, more use cases for HVDC will likely emerge as the energy transition reshapes power systems everywhere and highlights its role to preserve reliability and resiliency. Over the near term, HVDC’s primary role will be to provide balance and flexibility. “You’ll see tremendous interest in HVDC around the world for the recent efforts to integrate renewable resources that are typically in remote areas and bringing [that power] to the large load centers,” he predicted. “That is true for North America. It’s true for Europe. It’s true for the large systems in Asia. So, you’ll see a global interest for HVDC now that you have never seen in past history,” he said.
—Sonal Patel is a POWER senior associate editor (@sonalcpatel, @POWERmagazine).
The post The Vital Link: How HVDC Is Modernizing the Grid appeared first on POWER Magazine.